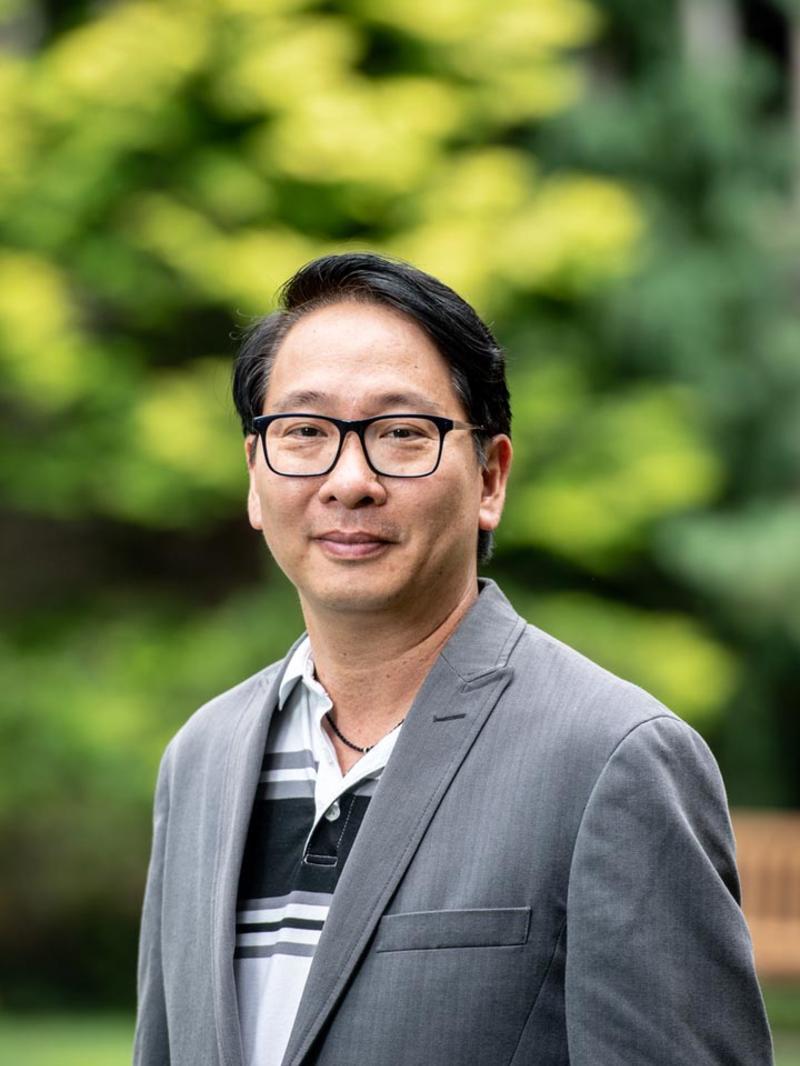
-
- BS in Chemical Engineering (1994), Rutgers University, NJ
- MS in Chemical Engineering (1997), University of Rhode Island, RI
- PhD in Chemical Engineering (2001), University of Rhode Island, RI
-
- Associate Professor (2016-present), Department of Biology, Saint Joseph‘s University, Philadelphia, PA
- Assistant Professor (2010-2016), Department of Biology, Saint Joseph‘s University, Philadelphia, PA
- Visiting Research Fellow (2009-2010), Technical University of Munich, Germany
- Assistant Research Scientist (2006-2009), Johns Hopkins University, Baltimore, MD
- Post-Doctoral Research Fellow (2002-2005), Johns Hopkins University, Baltimore, MD
-
- The pathogenic A391E mutation in FGFR3 induces a structural change in the transmembrane domain dimer. Mudumbi K†, Julius A, Herrmann J, Li E. Journal of Membrane Biology, 2013, 246(6):487-493.
- Increased expression of the integral membrane proteins EGFR and FGFR3 in anti-apoptotic Chinese hamster ovary cell lines. Ohsfeldt E, Huang SH, Baycin-Hizal D, Kristoffersen L, Le TMT, Li E, Hristova K, Betenbaugh MJ. Biotechnology and Applied Biochemistry, 2012, 59(3):155-162.
- Transmembrane helix dimerization: beyond the search for sequence motifs. Li E, Wimley W, Hristova K. Biochimica Biophysica Acta – Biomembranes, 2012, 1818(2):1698-1705.
- Assembly of the m2 tetramer is strongly modulated by lipid chain length. Schick S, Chen L, Li E, Lin J, Köper I, Hristova K. Biophysical Journal, 2010, 99(6):1810-1817.
- Receptor tyrosine kinase transmembrane domains: Function, dimer structure and dimerization energetics. Li E, Hristova K. Cell Adhesion and Migration, 2010, 4(2):249-254.
- Increased expression of the integral membrane protein ErbB2 in Chinese hamster ovary cells expressing the anti-apoptotic gene Bcl-xL. O'Connor S, Li E, Majors BS, He L, Placone J, Baycin D, Betenbaugh MJ, Hristova K. Protein Expression and Purification, 2009, 67(1):41-47.
- Utility of surface-supported bilayers in studies of transmembrane helix dimerization. Li E, Merzlyakov M, Lin J, Searson P, Hristova K. Journal of Structural Biology, 2009, 168(1):53-60.
- Quantitative measurements of protein interactions in a crowded cellular environment. Li E, Placone J, Merzlyakov M, Hristova K. Analytical Chemistry, 2008, 80(15):5976-85.
- Surface supported bilayer platform for studies of lateral association of proteins in membranes (Mini Review). Merzlyakov M, Li E, Hristova K. Biointerphases, 2008, 3(2):FA80.
- Effect of pathogenic cysteine mutations on FGFR3 transmembrane domain dimerization in detergents and lipid bilayers. You M, Spangler J, Li E, Han X, Ghosh P, Hristova K. Biochemistry, 2007, 46(39):11039-11046.
- Surface-supported bilayers with transmembrane proteins: role of the polymer cushion revisited. Merzlyakov M, Li E, Gitsov I, Hristova K. Langmuir, 2006, 22(24):10145-10151.
- Spectral Förster resonance energy transfer detection of protein interactions in surface-supported bilayers. Merzlyakov M, Li E, Casas R, Hristova K. Langmuir, 2006, 22(16):6986-6992.
- Role of receptor tyrosine kinase transmembrane domains in cell signaling and human pathologies. Li E, Hristova K. Biochemistry, 2006, 45(20):6241-6251.
- The achondroplasia mutation does not alter the dimerization energetics of the fibroblast growth factor receptor 3 transmembrane domain. You M, Li E, Hristova K. Biochemistry, 2006, 45(17):5551-5556.
- Transmembrane helix heterodimerization in lipid bilayers: probing the energetics behind autosomal dominant growth disorders. Merzlyakov M, You M, Li E, Hristova K. Journal of Molecular Biology, 2006, 358(1):1-7.
- Directed assembly of surface-supported bilayers with transmembrane helices. Merzlyakov M, Li E, Hristova K. Langmuir, 2006, 22(3):1247-1253.
- FGFR3 dimer stabilization due to a single amino acid pathogenic mutation. Li E, You M, Hristova K. Journal of Molecular Biology, 2006, 356(3):600-612.
- Sodium dodecyl sulfate-polyacrylamide gel electrophoresis and Forster resonance energy transfer suggest weak interactions between fibroblast growth factor receptor 3 (FGFR3) transmembrane domains in the absence of extracellular domains and ligands. Li E, You M, Hristova K. Biochemistry, 2005, 44(1):352-360.
- Imaging Förster resonance energy transfer measurements of transmembrane helix interactions in lipid bilayers on a solid support. Li E, Hristova K. Langmuir, 2004, 20:9053-9060.
- Pollution prevention guideline for academic laboratories. Li E, Barnett SM, Ray B. Journal of Chemical Education, 2003, 80:45-49.
- An alternative confirmatory test for silver ion in quantitative analysis. Kirschenbaum LJ, Resende E, Li E, Ruekberg B. Journal of Chemical Education, 2001, 78:1524.
- Application of temperature control strategies to the growth of hen egg-white lysozyme crystals. Schall CA, Riley JS, Li E, Arnold E, Wiencek JM. Journal of Crystal Growth, 1994, 165:299-307.
-
The main focus of my laboratory is to elucidate the physical and chemical principles behind the interaction of membrane proteins.
The plasma membrane (often referred as the cell membrane) is the structure that defines the cell, separating the extracellular (outside the cell) from the intracellular (inside the cell) environment. In addition to its function as a barrier, the plasma membrane allows the cell to sense changes in the extracellular space. Examples of these changes include variations in the concentration of small molecules (salt, sugar, etc.), changes in pH, presence of larger molecules (growth factors, pathogens, etc.), etc.
The two major components in a cell membrane are lipids and proteins. Lipids create a thin oily layer that separates the aqueous environment outside the cell from that inside the cell, while proteins are the ones that function as sensors of the outside environment. Proteins are often referred to as the workforce of the cell because they carry out much of the essential processes. They are large molecules composed of smaller units called amino acids. Although cells contain hundreds of different proteins, they are all made of a combination of only twenty amino acids. These twenty amino acids are very similar to each other, and only one part, referred to as the sidechain, distinguishes them. Thus, the physical and chemical properties of each amino acid is determined by its sidechain.
Although the size and structure of these membrane proteins may vary, a common feature is that they are embedded in the membrane, and are thus, referred to as transmembrane proteins. These transmembrane proteins have a portion in the extracellular environment, and another one inside the cell. The segment of the protein that is buried in the hydrophobic (oily) membrane is referred to as the transmembrane domain (TMD).
The research in my lab is centered on the transmembrane domains (TMDs) and membrane-proximal (juxtamembrane) segments of proteins that cross the membrane once (single-pass membrane proteins). The TMD of these proteins span the membrane in the shape of a helix (Figure 1). Studies of transmembrane domains lag behind those of the extracellular and intracellular domains, even though clinical studies have associated mutations in the TMDs with several genetic disorders.
The membrane proteins that my lab studies belong to a class of proteins that elicit the response to the extracellular signal by forming complexes with other membrane proteins. These complexes are often composed of two proteins interacting with each other, forming a stable new structure. Dimerization, therefore, is the process in which two monomers (single proteins) come together to form a dimer (Figure 2).
Cells, under normal conditions, must maintain a fine balance between monomers and dimers. Dimerization of membrane proteins is often one of the initial steps in a series of events that triggers cellular responses such as movement, division, and even cell death. Diseases in living organisms may arise because their cells cannot function properly if dimerization is out of control. The specific disease that may result depends on which membrane protein is affected. For example, unregulated dimerization of a membrane protein may result in unregulated cell growth and division, eventually leading to the formation of tumors. In some other cases, unregulated dimerization of another type of protein may lead to abnormalities during development such as dwarfism and cranial disorders. These are just a few examples highlighting the important physiological roles of these proteins and the medical relevance of studying membrane protein dimerization.